Bolocam
studies the Universe using the Sunyaev-Zel'dovich effect. 
Bolocam, a millimeter-wave
bolometer camera, was built to achieve two main science goals.
One was to learn more about galaxy and large-scale structure formation
via a blind survey for dusty galaxies (so called submillimeter
galaxies) and the other was to learn more about the dark matter and
dark energy that compose 96% of our universe via a blind survey for
clusters of galaxies using the Sunyaev-Zel'dovich effect
(SZE). Since it was designed as a survey instrument, the
field of view was made as large as possible while still providing
acceptable optical performance over the entire field. The detectors
were designed to have the best sensitivity possible, limited only by
the unwanted signal caused by the atmosphere above Mauna Kea.
Jack Sayers, a graduate student at Caltech, joined the Bolocam project
because the SZE survey provides a method to potentially place
high-precision constraints on the properties of dark matter and dark
energy. Since fall 2003, Sayers and his advisor, professor Sunil
Golwala, along with several of their colleagues at Caltech, JPL, CU
Boulder, and the University of Cardiff, have made a variety of
observations of the SZE using the CSO Bolocam, and Sayers was recently
awarded a PhD based the results of the CSO Bolocam SZE survey.
The SZE involves scattering of the oldest photons in the
universe, the cosmic microwave background radiation (CMBR), by the 10 -
100 million degree electron gas in clusters of galaxies. These
electrons are composed of 60 - 70% of the baryonic mass of the cluster,
compared to only 10 - 15% from the visible stars within the
cluster. Since the temperature of the CMBR is a few degrees above
absolute zero, these hot electrons increase the energy of the CMBR
photons. However, less than 1% of the CMBR photons are scattered by the
electrons, so the overall change in the spectrum of the CMBR is fairly
small. The magnitude of the change in the CMBR caused by the SZE is
proportional to the pressure of the electron gas multiplied by the size
of the cluster, typically a couple of Megaparsecs, which is more than
10 trillion miles or about 5 light years. Since the SZE increases the
energy of some CMBR photons, it basically takes some of the low energy
(low frequency) photons and moves them to higher energy (higher
frequency). The result is that the CMBR looks colder, or dimmer,
at a low frequency and hotter, or brighter, at higher frequencies.
Additionally, the magnitude of the SZE signal is the same no matter how
far away the cluster is, so it provides a method to study the oldest,
or most distant, clusters in the universe. By studying a large number
of these distant clusters it's possible to determine some of the global
properties of the universe, including the rate of expansion, the amount
of dark energy, and the amount of dark matter.
Bolocam was designed to observe at 143 GHz, which is near the
frequency where the maximum dimming of the CMBR due to the SZE occurs
(see Figure 1). This frequency is between the far infrared, which is
the thermal radiation absorbed by greenhouse gasses in the atmosphere,
and microwaves, which are used to cook food. At this relatively low
frequency the clusters appear as cold, or dim, spots compared with the
CMBR background in their maps. For the most massive clusters these cold
spots are less than 0.001 degrees different from the background CMBR,
which is about one part in 10000.
The first set of observations made were maps of "blank"
fields, or fields that contain no bright stars, galaxies, or other
objects. They mapped two fields, each one covering an area of 0.5
square degrees, or about twice the size of the moon. These observations
were surveys, to discover previously unknown clusters. To date, no one
has discovered a previously unknown cluster using the SZE. However,
there is still a signal in the map due to all of the unresolved
clusters that are too dim for them to be seen. To look for this signal
the angular power spectrum of their maps was determined, which shows
how the signal is distributed as a function of angular scale. This is
similar to determining the frequency spectrum of a musical instrument,
so you can find which notes it plays. They did not detect this power
spectrum signal, but they placed the first upper limit at 143 GHz for
these angular scales.
Their next set of observations focused on mapping the SZE
signal in known clusters. To date, there have been no published maps of
the SZE at 143 GHz, so these ongoing observations may produce the first
ones. Since the SZE directly measures the pressure of the electron gas,
it provides a fairly clean measurement of the cluster. Additionally,
compared to other observations of the cluster electron gas, the SZE
signal diminishes slowly when observing positions at small distances
away from the cluster center. Therefore, they can study the cluster
further away from its center. Their observations will help determine
the astrophysics that describe cluster formation, and provide a better
picture of how structure has formed and evolved in our universe.
Additionally, understanding the cluster astrophysics is critical to
being able to learn more about the global properties of our universe
from the distant clusters that will be discovered using the SZE in the
future. A Bolocam map of the cluster MS0451.6+0305 is shown in Figure 2.
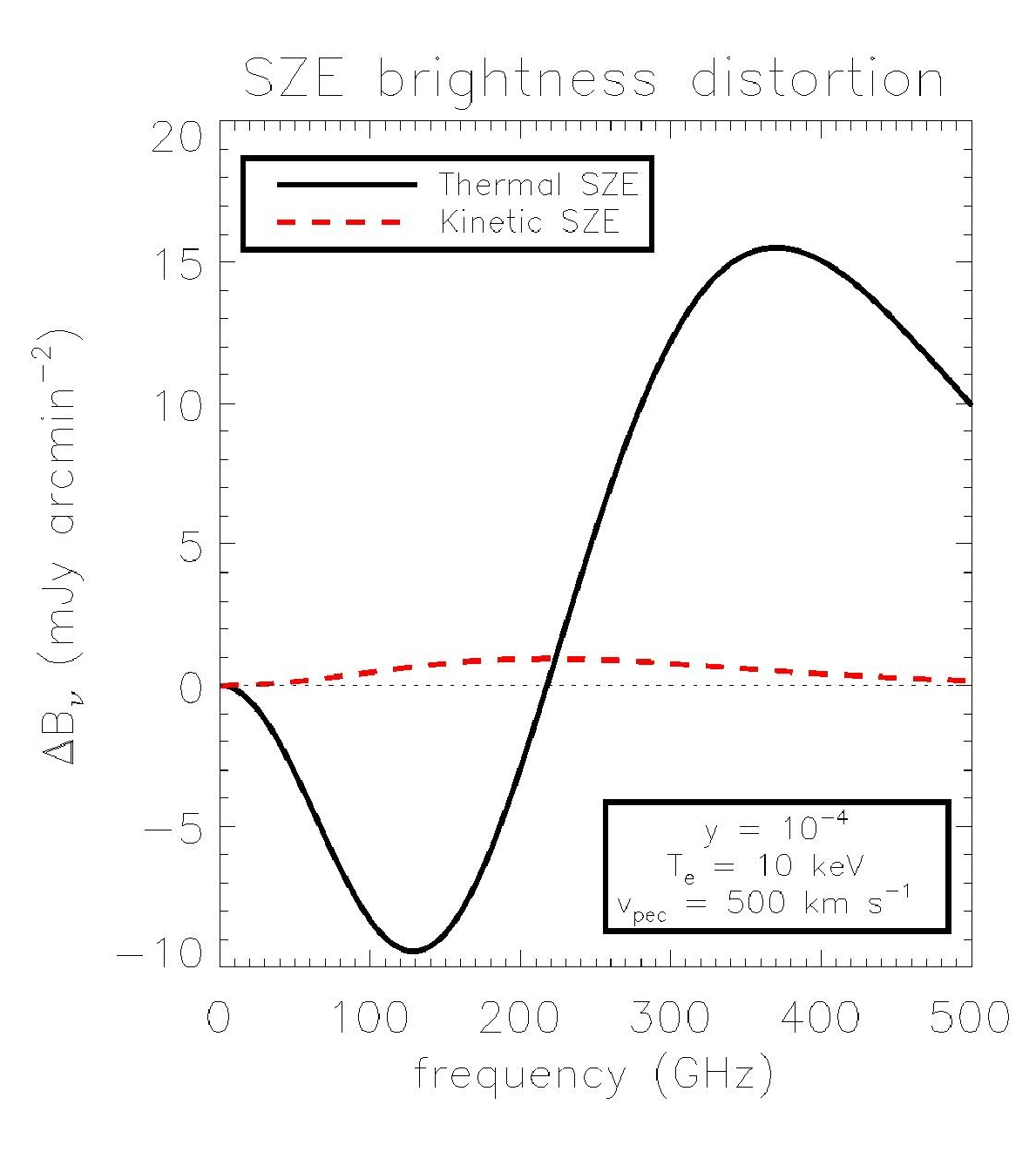
Figure 1. The relative distortion of the CMBR spectrum for a
typical massive cluster as a function of frequency.

Figure 2. Bolocam map of a massive galaxy cluster, MS0451.6+0305.
The plots shows the signal-to-noise ratio obtained by Bolocam, with
contours of S/N = 2, 4, and so forth. The central surface
brightness is about 0.0002 Kelvin, or more than 10000 times dimmer than
the CMBR.